Revista
Fuentes: El Reventón Energético
Vol.
15 Nº 1 de 2017 - Ene/Jun - pp 7/17
THE INFLUENCE OF CHEMICALS
ON ASPHALTENES PRECIPITATION:
A COMPARISON BETWEEN ATOMIC
FORCE MICROSCOPY AND NEAR
INFRARED
TECHNIQUES
Jeniffer
N. Figueira1;
Renata A. Simão1;
Bluma G. Soares1,2;
Elizabete F. Lucas1 , 2*
Universidade
Federal do Rio de Janeiro. Instituto de Macromoléculas – Av. Horácio Macedo.
2030, block J. Cidade Universitária. 21941598, RJ, Brazil.
Universidade
Federal do Rio de Janeiro, COPPE. Departamento de Engenharia Metalúrgica e de
Materiais. Av. Horácio Macedo, 2030, block F. Cidade Universitária. 21941598,
RJ, Brazil.
*E-mail:
elucas@metalmat.ufrj.br
ABSTRACT
Asphaltenes can cause
serious problems to petroleum industry. Indication of asphaltenes stability can
be obtained by precipitation induced by n-heptane, monitored by near-infrared
(NIR). However, this technique does not provide information on the particles
sizes. In this study, the precipitation onset of model systems (1wt%
asphaltenes in toluene) was monitored by NIR, with and without adding additive.
The particles sizes of the same systems were monitored by atomic force
microscopy (AFM): Dispersions of asphaltenes in toluene, n-heptane and mixtures
of these two solvents were analyzed by AFM. For the pure model system, the size
of the asphaltenes aggregates clearly increased in function of rising n-heptane
concentration in the solvent mixture, with this increase being much more
pronounced for solubility parameter values below that corresponding to the
precipitation onset. In the presence of
additives, significantly smaller particles were obtained even when the
precipitation onset showed only a slight shift.
Keywords: Asphaltenes,
Precipitation, Near Infrared Spectroscopy, Particle Size, Atomic Force
Microscopy.
LA
INFLUENCIA DE LOS PRODUCTOS QUÍMICOS EN LA PRECIPITACIÓN
DE
ASFALTENOS: UNA COMPARACIÓN ENTRE LAS TÉCNICAS DE
MICROSCOPÍA
DE FUERZA ATÓMICA Y DE INFRARROJO CERCANO
RESUMEN
Los asfaltenos pueden causar serios problemas en la industria de petróleo.
La evaluación de la estabilidad de los asfaltenos puede ser obtenida a través
de la precipitación inducida por n-heptano, monitoreada por infrarrojo cercano
(NIR). Sin embargo, esta técnica no provee información al respecto del tamaño
de partículas. En este estudio, el onset de precipitación de sistemas modelo
(asfaltenos en tolueno 1%p) fue monitoreado por NIR, con y sin la adición de
aditivo. Los tamaños de partículas de los mismos sistemas fueron monitoreados
por microscopía de fuerza atómica (AFM): dispersiones de asfaltenos en tolueno,
n-heptano y mezclas de estos dos solventes fueron analizadas por AFM. Para el
sistema modelo puro, el tamaño de los agregados de asfaltenos aumentó
claramente en función del aumento de la concentración de n-heptano en la mezcla
de solventes, siendo este aumento más marcado para valores de parámetros de
solubilidad menor que el correspondiente al onset de precipitación. En
la presencia de aditivos, partículas significativamente menores fueron
obtenidas aun cuando el onset de precipitación mostró sólo un ligero
cambio.
Palabras clave: Asfaltenos,
Precipitación, Espectroscopia Infrarrrojo Cercano, Tamaño de Partícula, Microscopía
de Fuerza Atómica.
Cita: Figueira, J.,
Simão, R. Soares, B. & Lucas, E. (2017). The influence of chemicals on asphaltenes precipitation: a comparison
between atomic force microscopy and near infrared techniques. Revista Fuentes: El reventón
energético, 15 (1), 7-17.
DOI: http://dx.doi.org/10.18273/revfue.v15n1-2017001
1.
INTRODUCTION
Asphaltenes are present in
the heaviest fraction of crude oil and are one of the main sources of problems
in the petroleum industry, because of their potential for precipitation and
consequent deposition as solid particles. This deposition can occur in various
stages, from the
start or production, by impeding flow inside the reservoir rock formation, to
the refining stage, by
clogging lines and fouling equipment. Besides this, asphaltenes are indicated
as the main reason for stabilization of water-in-oil emulsions. The
characteristics of the asphaltenes extracted depend on the origin of the oil and quantity/quality of
flocculant solvent
added during the separation process (Gray, 1994), (Mullins, Sheu, Hammami &
Marshall, 2007), (Ramalho, Lechuga & Lucas, 2010), (Honse, Ferreira,
Mansur, Gonzalez & Lucas,2012), (Honse, Mansur & Lucas, 2012),
(Marques, 2012).
The literature quotes two
spectroscopic methods to study the potential for precipitation of asphaltenes,
ultraviolet (UV) and near infrared (NIR). In both cases, the precipitation of
the asphaltenes is induced by adding a
flocculant solvent (an n-alkane with low molar mass, such as n-heptane). The absorbance
of the system is measured at a constant wavelength in function of the quantity of flocculant added to the system, and the formation of
asphaltenes aggregates can be detected by variation of the absorbance.
Asphaltenes are considered to be more stable the greater the quantity of flocculant that is required to
cause them to start
precipitating. This point is called the precipitation onset (Lucas,
Mansur, Spinelli & Queirós, 2009) (Garreto, Gonzalez, Ramos &Lucas,
2010) (Garreto, Mansur & Lucas, 2013) (Ferreira, Louzada, Gonzalez &
Lucas, 2015) (Ferreira, et al., 2016). The performance of chemical additives to inhibit deposition of
asphaltenes can also be evaluated by these techniques. A good additive should
shift the
precipitation onset to higher flocculant volumes, indicating their ability to increase the stability
of asphaltenes in the petroleum sample (Ferreira, et al., 2015) (Lima, Mansur,
Lucas & Gonzalez, 2010) (Lucas, Ferreira & Khalil, 2015) (Palermo &
Lucas, 2016).
Of these two spectroscopic
techniques, NIR is more comprehensive, because it allows analyzing model
systems and the crude oil itself, while the UV technique is only able to
analyze model systems of asphaltenes in toluene at low concentrations, because
at high concentrations the system becomes too dark to read the absorption
intensity (Garreto, et al., 2010) (Garreto, et al., 2013) (Ferreira, et al.,
2015) (Moreira, Lucas & Gonzalez, 1999) (Sousa, Oliveira, Lucas, González,
2004). On the other hand, the NIR technique to monitor the absorption intensity
of the system in function of flocculant
concentration, although more versatile, only provides information about the point where
the asphaltenes aggregates start to form, not the size of the particles formed.
It is known that the flow of crude
oil is influenced by
the formation of asphaltenes aggregates (Altoé, et al., 2014), and this influence depends on the size of the particles formed. In this
case, the evaluation of the performance of additives to stabilize asphaltenes
can produce false negatives, since the additive can present good performance in
reducing the size of the asphaltenes
aggregates without causing a significant shift in the precipitation onset.
The size of asphaltenes
aggregates can be monitored by measuring the light scattered (Rajagopal &
Silva, 2004) (Mansur, Melo & Lucas, 2012). However, this technique can only
be applied to model systems with very low asphaltenes concentrations, which
often does not allow studying model systems that reproduce the characteristics
of the crude oil of interest.
Atomic force microscopy
(AFM) is a technique that allows studying the morphology of materials with
atomic or molecular resolution (Weinsenhorn, Maivald, Butt & Hansma, 1992)
(Jagtap & Ambre, 2006), enabling its use to provide information about the
aggregative character of asphaltenes molecules. Even though knowledge of the
morphology of asphaltenes is scant and the AFM technique is a powerful tool for
this type of study, few articles have been published in this respect (Toulhoat,
Prayer & Rouquet, 1994) (Loeber, Muller, Morel & Sutton, 1998) (Long,
Xu & Masliyah, 2007) (Madge & Garner, 2007) (Syunyaev,
Balabin, Akhatov &Safieva, 2009)
(Wang, Liu, Zhang, Masliyah
&Xu, 2010) (Pizzorno, 2010) (Natarajan, 2011) (Wang, et al., 2012)
(Natarajan, et al., 2014). The most important exceptions that can be mentioned
are: (i) the formation of complex asphaltenes structures dispersed in the
maltene matrix in asphaltic cement (Toulhoat, et al., 1994) (Pizzorno, 2010);
(ii) the adsorption of asphaltenes on oil reservoir rocks, which can partially or totally block the
oil’s flow through the
porous medium (Syunyaev, et al., 2009); and (iii) the characteristics of the
asphaltenes aggregates formed in function of the interactions that occur with
solvents having varied polarities (Madge & Garner, 2007) (Natarajan, 2011).
Therefore, AFM is still little explored as a complementary technique to NIR or
UV spectroscopy to assess the stability of asphaltenes and the performance of
chemical additives, by monitoring the particle size in function of the
solubility parameter (δ) of solvent mixtures, as is done
through titration of n-heptane
and monitoring the absorption by NIR. In this article, we report a systematic
study to evaluate the particle sizes of asphaltenes by AFM in mixtures of
solvents (solvent + non-solvent), mainly with solubility parameters near the
precipitation onset as determined by NIR, to monitor the aggregative behavior
of asphaltenes during the precipitation process, with and without the presence
of a stabilizing additive.
2.
EXPERIMENTAL
2.1. CHARACTERIZATION OF C7IP
ASPHALTENES BY FOURIER-
TRANSFORM INFRARED
(FTIR) SPECTROSCOPY
The C7IP asphaltenes
sample, donated by Petrobras, was characterized by FTIR using the casting film technique in a KBr cell containing the
dispersion of asphaltenes in toluene, in the region between 4000 and 400 cm-1, with resolution of 4 cm-1at room temperature, in a Varian
Excalibur FTS 3100 spectrometer. This technique is very useful to identify the
main functional groups, by obtaining the complete vibrational spectrum of the molecule of interest and confirming
the asphaltenes fraction
of the sample.
2.2. DETERMINATION OF THE
ASPHALTENE PRECIPITATION
ONSET BY NEAR INFRARED
(NIR) SPECTROSCOPY
We first prepared a model
dispersion of 1wt% asphaltenes in toluene in a round-bottom flask. When an additive
was included, it was weighed and placed in the same beaker. Then the beaker was
filled to the top with toluene, placed in an ultrasound bath for 15 minutes and
left at rest for 24h. The asphaltenes precipitation onset was determined in a
Bruker Matrix-F NIR spectrometer. The analysis was carried out by adding a
non-solvent (n-heptane) to the system containing the asphaltenes. In this
process, 10 mL of the model system was placed in a conical receptacle, into
which the external probe of the spectrometer (optical path of 5 mm) was
inserted. Then an isocratic pump was used to add n-heptane at a flow of 2
mL/min. The device was programmed to take absorbance readings at 1600 nm in
function of the volume of n-heptane added to the system (Garreto, et al., 2010)
(Garreto, et al., 2013). The initial addition of n-heptane causes dilution of
the system, and thus reduction of the absorbance. When the n-heptane volume is
large enough to cause the asphaltenes to precipitate, the formation of the
resulting aggregates causes an increase in absorbance. Therefore, the volume of
n-heptane added to 1 mL of the model system of asphaltenes necessary to
increase the absorption is taken to be the precipitation onset (Syunyaev, et
al., 2009) (Pizzorno, 2010. In practice, the volume of n-heptane at which the
absorbance value is lowest is observed (Natarajan, 2011) (Henriques, Winter,
Koroishi, Maciel & Bueno, 2011).
2.3. CHARACTERIZATION OF
ASPHALTENE PARTICLE SIZE BY
ATOMIC FORCE MICROSCOPY (AFM)
Unlike analysis of the
precipitation onset by NIR, with AFM it is not possible to carry out a
titration procedure, making it necessary to prepare various systems containing diverse toluene:heptane
(solvent:flocculant) ratios.
In a preliminary study, we prepared dispersions of asphaltenes in toluene and
in heptane at a concentration of about 0.125 g/L. Two mica sheets, previously
washed with isobutyl alcohol, were used, each one placed inside the
dispersions. Then the mica sheets were left to dry in an exhaust hood and
observed by AFM. For the other analyses, the dispersions were prepared with
1wt% of asphaltenes in the solvent for evaluation (toluene, heptane or a
mixture of the two), placed in an ultrasound bath for 15 minutes and then left
at rest for 24h in the dark. The previously cleaned mica sheets were immersed
in the dispersions. The toluene:heptane ratios used were chosen in function of
the precipitation onset determined by NIR, i.e., the ratios related to the
precipitation onset point and just below and above it. Two mica sheets were
prepared for each dispersion.
The mica sheets were
analyzed under a JPK NanoWizard AFM, operating in non-contact mode at room
temperature, using a Bruker RTESPA probe, with resonance frequency of 328 to
388 KHz and spring constant of 20-80 N/m, for imaging at a scan rate of 0.8 Hz.
All the images had size of 10µm x 10µm. The images were analyzed using the JPK
Image Processing program, by
examining an average of five regions, and the results were expressed in tables presenting the maximum sizes indicated at the side
of each figure, which
corresponds to the maximum particle size in one of its dimensions.
RESULTS
AND DISCUSSION
3.1. CHARACTERIZATION OF
C7IP ASPHALTENES BY FTIR
In this work we used two
types of asphaltenes samples, one extracted from petroleum and the other from refinery asphaltic residue,
identified as C7IP and C7IR, respectively. Sample C7IP was characterized by FTIR to confirm the asphaltenes (Figure 1).
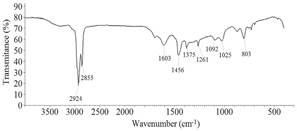
Figure 1. FTIR spectrum of asphaltenes C7IP
Absorption bands could be
observed at 2924 and 2855 cm-1, indicating axial stretching
vibrations of CH3 groups; a band at 1603cm-1, characteristic of C=C stretching vibrations; C-H vibrations at 1456 cm-1 and 1375 cm-1, attributed respectively to
asymmetric and symmetric deformations of methyl groups; a band at 803 cm-1, possibly related to the C-H deformation of aromatic groups; and
absorption bands between 3472 and 3413 cm-1,
posibly corresponding to axial C-H vibrations of sp2 carbons of the aromatic groups. These same bands were observed in a
previous study involving the C7IR asphaltene sample (Palermo & Lucas,
2016).
3.2. DETERMINATION OF
ASPHALTENE PRECIPITATION
ONSET BY N-HEPTANE
TITRATION USING NIR
In the precipitation onset
test using NIR, the absorption intensity decreases by the effect of dilution
caused by addition of n-heptane. When the quantity of n-heptane becomes sufficient to induce
formation of molecular aggregates,
the absorption intensity starts to increase. Therefore, the precipitation onset is identified as the minimum absorption intensity
value, and can be reported in terms of mL of n-heptane/mL of asphaltenes dispersion.
We obtained precipitation
onset results for the C7IP asphaltenes sample from absorbance curves in the
near infrared (NIR) region in function of volume of n-heptane added to the
model system (1 wt% asphaltenes in toluene), with and without a chemical
additive. The purpose of these measurements was to observe the shift of the
precipitation onset in function of the presence of a chemical additive and to
establish the toluene:nheptane ratios to be used in preparing the dispersions
for analysis of the asphaltenes particle sizes by AFM.
Figure 2
presents the absorption intensity curves according to the volume of n-heptane
added to 10 mL of the dispersion of C7IP asphaltenes in toluene. The test
without the presence of stabilizer was performed in duplicate. It can be seen
that the results were reproducible, with precipitation onset of asphaltenes in
1mL of n-heptane/mL of asphaltenes dispersion. This result also can be
expressed in terms of a TOL:HEP ratio of 50:50 v/v. Based on an empirical
scale, this model system could be considered unstable. The addition of 0.01 wt%
of copolymer shifted the precipitation onset to a slightly higher value (1.1 mL
of n-heptane/ mL of asphaltenes dispersion). This result is the same as TOL:HEP
ratio of 48:52 v/v. This indicates that the additive, at the concentration
employed, did not perform satisfactorily in terms of shifting the precipitation
onset. This is an interesting result, because it shows that an additive that has weak influence on
the precipitation onset
can affect the particle size of the aggregates formed. The tests were run in
duplicate with an error of 0.05 mL of
n-heptane/mL of model dispersion.
We also performed these
tests for the model system at 1 wt% of C7IR asphaltenes in toluene, with and
without addition of cardanol. The precipitation onset results are summarized in
Table 1 together with the results obtained previously for the C7IR asphaltenes
model system, with and without addition of the selected copolymer (Palermo
& Lucas, 2016).
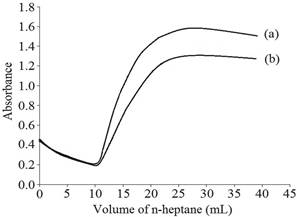
Figure 2. Absorbance at 1600 1600 cm-1 as a function of volume of n-heptane added to 10 mL of C7IP asphaltenes
dispersion in toluene (a)
and to the same dispersion containing 0.01 wt% of copolymer (b)
Table 1. Asphaltenes precipitation onset (mL of
n-heptane/ mL of asphaltenes dispersion) with and without additive (Palermo
& Lucas, 2016).
Asphaltenes fraction
|
Asphaltenes precipitation onset (mL of n-heptane/mL of asphaltenes
dispersion)
|
|
Copolymer (wt%)
Cardanol (wt%)
|
|
No additive 0.01 0.10 0.30
|
C7IP 1.00 1.10 - -
C7IR
|
1.30*
|
1.80*
|
1.35
|
1.60
|
We also analyzed the
effect of adding cardanol to the C7IR system on the change in the precipitation
onset. The addition of
0.1 wt% of cardanol did not cause a significant variation in the onset value. However, adding
0.3 wt% of cardanol shifted the onset to 1.6 mL of n-heptane/mL of model
dispersion, an increase of 15.4%. This result demonstrates the effectiveness of
cardanol as a stabilizer, as previously reported (Moreira, et al., 1999).
However, the
copolymer presented similar results at significantly lower concentrations for the C7IR model system.
The results presented in
Table1, some with and some without influence
on the asphaltenes precipitation onset, are very interesting to investigate the asphaltenes particle
sizes, in each case. This was done here by atomic force microscopy.
3.3. DETERMINATION OF
ASPHALTENE PARTICLE SIZE BY
ATOMIC FORCE MICROSCOPY (AFM)
As mentioned, information
on the phase behavior of asphaltenes under different conditions of composition,
temperature and pressure is very important to resolve problems found during the
production, transport and refining of
oil. The stability of asphaltenes can be inferred by spectroscopic tests with variable
addition of a
flocculant solvent, most commonly n-heptane. Spectroscopic techniques can indicate the
volume of n-heptane necessary to start the precipitation of asphaltenes, but
they do not provide information on the size of the particles formed. Therefore,
relying only on these techniques alone to assess the performance of an additive
can give misleading results, such as indicating total inefficiency for failing to shift the
precipitation onset
even when the additive induces the formation of smaller aggregates. AFM was
used to observe the sizes of the asphaltenes particles formed in function of
adding the
flocculant (n-heptane) and the chemical additives. Since we did not find any
description in the literature of tests employing AFM for this purpose, it was necessary to carry out
preliminary assays to establish the methodology.
Mica is a standard
substrate used in AFM because it has an extremely flat surface with high
chemical stability and little influence on the film that forms on it (Liu &
Wu, 2000). Therefore, the first images obtained were of mica sheets only washed
in alcohol (Figure 3a) and sheets
immersed in toluene (Figure 3b) and
n-heptane (Figure 3c), since these
were the two solvents used in the tests of the model systems containing
asphaltenes. The maximum heights detected were around 30 nm, evidencing that
values near or below this can be related to the mica sheet itself instead of
the asphaltenes particles.

Figure 3. AFM images of pure mica washed with alcohol
(a) and after being immersed in toluene (b) and n-heptane (c)
Then we obtained images of
mica sheets submersed in the dispersion of C7IP asphaltenes in toluene (Figure 4a) and in n-heptane (Figures 4b
and 4c), after
leaving the dispersion to rest for 24 hours. By visual observation, the
dispersion of asphaltenes in toluene was homogeneous and very dark, while that
in n-heptane was heterogeneous, with sediment particles at the bottom of the
beaker. The AFM images agreed with the visual inspection. The mica immersed in
the dispersion of asphaltenes in toluene (Figure 4a) had the largest particle sizes on
about 18 nm. This value is comparable with those observed for mica alone,
indicating that the asphaltenes particle sizes were smaller than or equal to
this value. Values on the order of 10 nm have been reported in the literature,
obtained by light scattering measurements (Mansur, et al., 2012) for
dispersions of asphaltenes in a good solvent (toluene), when there is good
interaction between solute and solvent. This corroborates the result obtained
by AFM for this system. On the other hand, in Figures 4b and 4c, since
asphaltenes in n-heptane generate a heterogeneous dispersion, larger aggregates
can be observed, respectively of 100 and 1000 nm, because n-heptane is not a
solvent, so it was showed preferentially asphaltenes-asphaltenes interactions.
The differences
observed in these two figures are related to differences in the procedure for preparing the
two mica sheets.
The image in Figure 4b was obtained after immersion of the
mica in the dispersion allowed to rest for 24 h. In this case, the aggregates
observed visually (those with sizes
greater than 100 nm), were in the flask’s bottom sediment, so those collected on the mica
were not representative of the average particle sizes of the aggregates in this
system. In contrast, in the image in Figure 4c the mica was immersed in the
dispersion while under agitation to assure a representative range of particle
sizes in the medium would be collected. The particle sizes observed are around
1,000 nm, in agreement with the values mentioned in the literature for model
systems of asphaltenes in toluene:heptane mixtures (with high heptane content) with
significantly lower
asphaltenes concentrations, analyzed by light scattering (Mansur, et al.,
2012). These results indicate the need to immerse the mica sheet in the
dispersion while under agitation to obtain a representative particle size
reading. These results also demonstrate that, even in heptane, less polar
asphaltenes molecules formed relatively small aggregates (110 nm).
The
proportions of the toluene-heptane mixtures (TOL:HEP) for preparing the
asphaltenes dispersions at
1.0 wt% were chosen based
on the precipitation onset results obtained by NIR. For the C7IP asphaltenes,
the onset was 1.0 mL of n-heptane/mL of model system, corresponding to a
TOL:HEP ratio of 50:50 v/v. Therefore, we established lower TOL:HEP ratios,
near or above the precipitation onset, which were 71:29, 65:35 , 60:40, 55:45,
50:50, 42:58 and 29:71 v/v.
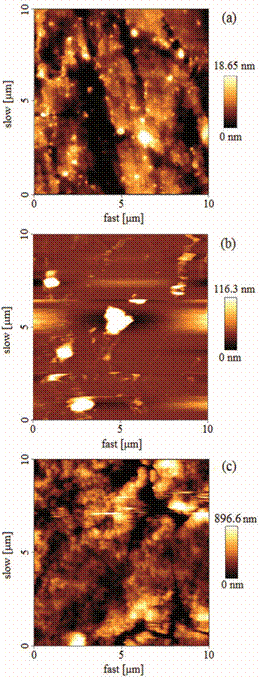
Figure 4. AFM images of the mica surface after being
immersed into the C7IP asphaltenes dispersion in: (a)
toluene, (b) n-heptane
under resting and (c) n-heptane under stirring.
The results are summarized
in Table 2, which presents the maximum
size identified to the right of each image. The different values for the same system
correspond to different observed areas. Figure 5 presents some of the images
captured during the experiment. A general analysis of the data in Table 2 shows coherent results, since the
particle sizes are considerably larger than those observed starting at the
TOL:HEP ratio of 55:45 v/v, slightly
lower than the precipitation onset identified by the NIR technique (TOLT:HEP of 50:50). In this
respect, precipitation onset results of titration with n-heptane, obtained by
optical microscopy (Garreto, et al., 2010) (Garreto, et al., 2013) (Ferreira,
et al., 2015) (Ferreira, et al., 2016), indicate that the start of precipitation
occurs with addition of a slightly lower volume of n-heptane than that identified by the minimum
absorbance point. Therefore, it is coherent to observe a significant increase in the asphaltenes particle size,
corresponding to the formation of asphaltenes aggregates, in lower n-heptane
volumes than that of the onset determined by NIR.
Table 2. Maximum sizes of C7IP asphaltenes particles
dispersed in different composition of toluene:n-heptane
(TOL:HEP), in each area
observed by AFM (values were taken from the indicator at the right side of each
image)
TOL:HEP
(v:v)
|
Maximum
sizes in each observed area*
( nm )
|
100:0
|
18
|
37
|
22
|
71:29
|
21
|
44
|
-
|
65:35
|
54
|
30
|
-
|
60:40
|
34
|
42
|
-
|
55:45
|
355
|
119
|
-
|
50:50
|
68
|
100
|
|
42:58
|
454
|
112
|
-
|
29:71
|
865
|
390
|
345
|
* Different values, for
the same system, correspond to different observed areas
It is important to note
that the interpretation of the analyses can vary according to the area chosen
for observation, mainly for systems containing higher n-heptane content (above
the precipitation onset of asphaltenes), as indicated by the size variation,
for each system, presented in Table 2. This is due to the polydisperse nature
in terms of polarity of the fractions extracted from the crude oil. The polydisperse nature can be observed in the AFM
images (a single image shows a
significant variation of particle sizes).
Besides this, for
n-heptane concentrations higher than 45 vol%, the size of the aggregates tends
to increase with rising n-heptane content. The results for the TOL:HEP ratio of
29:71 (223 – 865 nm) indicate the need for careful preparation of the mica
sheets, since substantially smaller particles (150 nm) were obtained when the
sheets were immersed in the system after sedimentation.
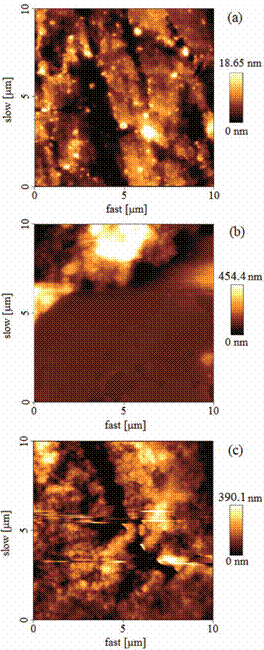
Figure 5. AFM images of the mica surface after being
immersed into the C7IP asphaltenes dispersed in TOL:HEP mixture 100:0 (a),
42:58 (b) and 29:71 (c)
Comparing the results
obtained for the TOL:HEP proportions of 100:0, 71:20, 65:35 and 60:40v/v, it is
suggested that the particle sizes in these systems are equal, taking into
consideration that the asphaltenes are also polydisperse in relation to their
particle size.
Therefore, it would be
necessary to observe different areas, a procedure that was not adopted for all
the systems analyzed, such as that with asphaltenes in pure toluene, for which
only one place on the mica sheet was observed. At the start of precipitation,
there are still many asphaltenes particles with the same sizes as those
dispersed in a good solvent (pure toluene).
Table 3
presents the compilation of the maximum sizes observed in each AFM image of the
mica sheets immersed in the TOL:HEP 50:50, 45:55 and 42:58 v/v solvent systems,
containing 1 wt% of asphaltenes, with copolymer addition of 0.01 wt%. The
different values for the same system correspond to different observed areas. The precipitation onset
identified by NIR for the
system of C7IP asphaltenes in toluene containing 0.01 wt% of copolymer was 1.10
mL of n-heptane/ mL of asphaltenes dispersion, which corresponds to a TOL:HEP
ratio of 48:52v/v. The shift of the onset caused by the additive’s action was +
0.1 mL of n-heptane/mL of asphaltenes dispersion. The addition of 0.01 wt% of
copolymer to the dispersion promoted the formation of particles with relatively
small sizes due to the addition of higher n-heptane volumes, which is coherent
with the slight shift in the precipitation onset to a higher value identified by the NIR technique. The
AFM images show that
even for heptane concentrations slightly higher than that corresponding to the
precipitation onset, the particle sizes remain relatively small. A slightly more significant increase in particle sizes,
characterizing the aggregation process, is observed for higher heptane
concentrations.
Table 3. Maximum sizes of the C7IP asphaltenes
particles dispersed in different compositions of toluene:n-heptane (TOL:HEP) in
the presence of 0.01 wt% of copolymer, in each area observed by AFM (values
were taken from the indicator at the right side of each image)
TOL:HEP
(v:v)
|
Maximum sizes in each obs
(nm)
|
erved
area*
|
50:50
|
30
|
33
|
43
|
65 -
|
45:55
|
23
|
17
|
106
|
- -
|
42:58
|
384
|
92
|
221
|
197 73
|
* Different values, for
the same system, correspond to different observed areas
Continuing the particle
size investigation by AFM of the systems previously evaluated by NIR (Table1),
we performed tests with the C7IR asphaltenes without and with addition of 0.1
or 0.3 wt% of cardanol in solvent systems with TOL:HEP proportions of 100:0,
48:52, 43:57, 40:60 and 35:65 v/v and 1 wt% of asphaltenes. Table 4 reports the
maximum particle sizes observed in each AFM image. Figure 6 presents some AFM images from which
the maximum sizes were obtained.
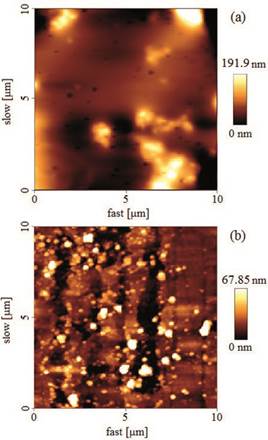
Figure 6. AFM images of the mica surface after being
immersed into the C7IR asphaltenes dispersed in TOL:HEP
(40:60) mixture: (a)
without adding cardanol, and (b) with adding 0.3 wt% of cardanol.
It should be recalled that
the TOL:HEP ratios for which the precipitation onset values were detected by
NIR were 43:57, 43:57 and 38:62 v/v, respectively, for the C7IR asphaltenes
without cardanol, with 0.1 wt% cardanol and 0.3 wt% cardanol, which correspond
to the onset values presented in Table 1.
The results in Table 4 show, for the system without additive, an increase
in the particle size with increased n-heptane content that corresponds to
points above the onset point, as expected. With addition of 0.1 wt% cardanol,
the sizes remained relatively small, even for proportions above the onset
level. These sizes continued being significantly smaller when using 0.3 wt%
cardanol for the entire range of proportions analyzed. Therefore, specifically
for the cardanol concentration of 0.1 wt%, we suggest that the additive acted
to reduce the particle size, even though the precipitation onset (determined by
using NIR to observe the variation of absorbance caused by titration with
n-heptane) did not vary significantly in relation to the test with the additive’s presence: from
1.30 to 1.35 mL of n-heptane/mL of asphaltenes dispersion in toluene.
These results suggest that the observation of the efficiency of additives
by the NIR technique can, in some cases,
lead to mistaken conclusions, since the problem of asphaltenes precipitation
during production of petroleum is directly related to the size of the
aggregates formed. Based on the AFM results, the additive in question would
present better performance than that identified by NIR spectrometry.
Table 4. Maximum sizes of
the C7IR asphaltenes particles dispersed in different compositions of
toluene:n-heptane
(TOL:HEP) in the
presence of 0.1 and 0.3 wt% of cardanol, in each area observed by AFM (values
were taken from the
indicator at the right side of each image)
TOL:HEP v:v
|
|
|
|
Highest size at each AFM image (nm)
|
|
|
No additive
|
|
0.1 wt% of cardanol
|
0.3
wt% of cardanol
|
100:0
|
2
|
3
|
23
|
27 104 134
|
31 13 5
|
48:52
|
74
|
147
|
16
|
15
|
314
|
188
|
53
|
86
|
73
|
43:57
|
12
|
37
|
24
|
47
|
77
|
-
|
5
|
9
|
3
|
40:60
|
205
|
192
|
-
|
19
|
28
|
18
|
68
|
77
|
106
|
35:65
|
272
|
254
|
300
|
173
|
129
|
135
|
31
|
7
|
8
|
|
|
|
|
|
|
|
|
|
|
|
|
|
4. CONCLUSIONS
Atomic force microscopy
(AFM) is a promising tool to evaluate the size of asphaltenes particles in
function of the characteristics of the solvent used, including identification of the formation of
molecular aggregates, in systems
with relatively high asphaltenes concentrations, overcoming a drawback of light
scattering to determine particle sizes. The results provided by AFM are more
realistic regarding the dispersion of asphaltenes in solvent media than those
supplied by near infrared (NIR) spectrometry to monitor the precipitation
onset. In the
presence of additives, we found that significantly smaller particle sizes can occur even with a
very small shift in the precipitation onset. Therefore, AFM can be used as a
complementary technique to select the best type and concentration of
stabilizing additives for application in the petroleum industry.
However, it is important
in AFM to observe a large number of areas, to obtain more representative
information on the system as a whole, and the interpretation of the shape of
the aggregates requires varied
magnification of the images, since the particles’ size can vary from 10nm to 10000 nm. Finally,
the use of a data treatment program is very useful in provide information on
the particle size distribution, and further research is in progress taking in
account this parameter.
5. ACKNOWLEDGEMENTS
The authors thank the
Brazilian agencies (CNPq, CAPES,
FAPERJ and ANP) for the financial support, and Petrobras for the donation of the asphaltic
residue samples.
6. REFERENCES
·
Altoé, R., Oliveira, M. C. K., Lopes, H. E.,
Teixeira, C., Cirilo, L. C. M., Lucas, E. F., Gonzalez, G. (2014). Solution behavior of asphaltic
residues and deasphalted oil prepared by extraction of heavy oil. Colloid Surf A, 445,
59-66.
·
Ferreira, S. R., Barreira, F. R., Spinelli, L.,
Seidl, P., Leal, K. Z., Lucas, E. F. (2016). Comparison between asphaltenes (sub)fractions extracted from two different
asphaltic residues: chemical characterization and phase behavior. Quim Nova, 39, 26-31.
·
Ferreira, S. R., Louzada, H. F., Gonzalez, G.,
Lucas, E. F. (2015). Influence of
the architecture of additives
on the stabilization of asphaltenes and water-in-oil emulsions separation. Energy Fuel; 29:
7213-7220.
·
Garreto, M. S. E., Gonzalez, G., Ramos, A. C.,
Lucas, E. F. (2010). Looking
for a model solvent to disperse asphaltenes. Chem Chem
Technol, 4,
317-323.
·
Garreto, M. S. E., Mansur, C. R. E., Lucas, E.
F. (2013). A model system to
assess the phase behavior of asphaltenes in crude oil. Fuel, 113, 318-322.
·
Gray,
M.R. (1994). Upgrading Petroleum Residues and Heavy Oils. New York: Marcel Dekker.
·
Henriques, C. B., Winter, A., Koroishi, A. T.,
Maciel Filho, R., Bueno, M. I. M. S. (2011). Estudo da
influência dos particulados no fenômeno de agregação dos asfaltenos por
espectrometria de varredura óptica. Quim
Nova, 34, 424-429.
·
Honse, S. O., Ferreira, S. R., Mansur, C. R. E.,
Gonzalez, G., Lucas, E. F. (2012). Separation and characterization of
asphaltenic subfractions.
Quim Nova, 35, 1991-1994.
·
Honse, S. O., Mansur, C. R. E., Lucas, E. F.
(2012). The
influence of asphaltenic subfractions on the stability of crude oil model emulsions. J Braz Chem Soc, 23,
2204-2210.
·
Jagtap, R. N., Ambre, A. H. (2006). Overview literature on atomic force
microscopy (AFM): basics and its important applications for polymers
characterization. Ind
J Eng Mat Sci, 13, 368-384.
·
Lima, A. F., Mansur, C. R. E., Lucas, E. F.,
Gonzalez, G. (2010). Polycardanol
or sulfonated
polystyrene
as flocculants for asphaltene dispersions. Energy
Fuels, 24, 2369-2375.
·
Liu, A., Wu, R. C., Eschenazi, E., Papadopoulos,
K. (2002). AFM on humic acid
adsorption on mica. Colloids
Surf A, 174, 245-252.
·
Loeber,
L., Muller, G., Morel, J., Sutton, O. (1998). Bitumen in colloid science: a
chemical, structural and rheological approach. Fuel, 77, 1443-1450.
·
Long, J.,
Xu, Z., Masliyah, J. H. (2007). Single Molecule Force Spectroscopy of Asphaltenes
Aggregates. Langmuir;
23: 6182-6190.
·
Lucas, E.F., Ferreira, L. S., Khalil, C. N.
(2015).
·
Polymers Applications in Petroleum
Production, In Encyclopedia of Polymer Science and Technology. Ed. H. F. Mark. New
York:John Wiley & Sons.
·
Lucas EF, Mansur CR, Spinelli L, Queirós YGC. (2009). Polymer science applied to
petroleum production. Pure
Appl Chem, 81, 476-494.
·
Madge
DN, Garner WN. (2007). Theory of asphaltene precipitation in a hydrocarbon
cyclone. Miner
Eng, 20, 387-394.
·
Mansur, C. R. E., Melo, A. R., Lucas, E. F.
(2012). Determination of asphaltenes
particles size: Influence of flocculant, additive and temperature. Energy Fuels 26,
4988.
·
Marques, L. C. C., Pereira, J.O., Bueno, A. D.,
Marques, V. S., Lucas, E. F., Mansur, C. R. E., Machado, A. L. C., González, G.
(2012). A study of asphaltenes-resins interactions. J Braz
·
Chem
Soc, 23, 1880-1888
·
.
·
Moreira, L. F. B., Lucas, E. F., Gonzalez, G.
(1999). Stabilization of
asphaltenes by phenolic compounds extracted from cashew-nut shell liquid. J Appl Polym Sci, 73,
29-34.
·
Mullins,
O. C., Sheu, E. Y., Hammami, A., Marshall, A. G. (2007). Asphaltenes, heavy oils, and
petroliomics. New York:Springer.
·
Natarajan,
A., Kuznicki, N., Harbottle, D., Masliyah, J., Zeng, H., Xu, Z. H. (2014). Understanding Mechanisms of Asphaltene
Adsorption from Organic Solvent on Mica. Langmuir, 30, 9370-9377.
·
Natarajan, A., Xie, J., Liu, S. W.,
Masliyah, J., Zeng, H., Xu, Z. (2011). Understanding Molecular Interactions of
Asphaltenes in Organic Solvents Using a Surface Force Apparatus. J Phys Chem, 115,
16043-16051.
·
Palermo, L. C. M., Lucas, E. F. (2016). Asphaltene aggregation: influence of composition of copolymers based on
styrene-stearyl methacrylate and styrene-stearylcinnamate containing sulfate
groups. Energy
Fuels, 30, 3941-3946.
·
Pizzorno, B. S. (2010). Efeito do solvente e da temperatura na morfologia
superficial do cimento asfáltico de petróleo. Instituto Alberto Luiz
Coimbra de Pós-Graduação e Pesquisa em Engenharia, Universidade Federal do Rio
de Janeiro: Rio de Janeiro.
·
Rajagopal, K., Silva, S. M. C. (2004). An experimental study of asphaltene
particle sizes in n-heptane-toluene mixtures by light scattering. Braz J Chem Eng, 21,
601-609.
·
Ramalho, J. B. V. S., Lechuga, F. C., Lucas, E.
F. (2010). Effect of the structure
of commercial poly(ethylene oxide-b-propylene oxide) demulsifier bases on the demulsification of water-in-crude oil emulsions:
Elucidation of the
demulsification mechanism. Quim Nova, 33, 1664-1670.
·
Sousa, M. A., Oliveira, G. E., Lucas, E. F., González,
G. (2004). The onset of
precipitation of asphaltenes in solvents of different solubility parameters. Progr Colloid Polym Sci,
128, 283287.
·
Syunyaev, R.
Z., Balabin, R. M., Akhatov, I. S., Safieva, J. O. (2009). Adsorption of
petroleum asphaltenes
onto reservoir rock sands by nearinfrared (NIR) spectroscopy. Energy Fuels, 23,
1230-1238.
·
Toulhoat,
H., Prayer, C., Rouquet, G. (1994). Characterization by atomic force
microscopy
·
of
adsorbed asphaltene. Colloids Surf A, 91, 267-283.
·
Wang, S.,
Liu, J., Zhang, L., Masliyah, J., Xu, Z. (2010). Interaction Forces between Asphaltene
Surfaces in Organic Solvent. Langmuir,
26, 183-190.
·
Wang, J.,
Opedal, N., Lu, Q., Xu, Z., Zeng, H., Sjoblom, J. (2012). Probing
Molecular Interactions of an
Asphaltene Model Compound in Organic Solvents Using a Surface Forces Apparatus
(SFA). Energy
Fuels, 26, 2591-2599.
·
Weinsenhorn,
A. L., Maivald, P., Butt, H–J., Hansma, P. K. (1992). Measuring adhesion,
attraction, and repulsion between surfaces in liquids with an atomic-force
microscope. Phys
Rev B, 45, 11226-11232.